Authors: Jiangui Liu, Tim Martin
Status: National Coverage 1981 to 2016
Summary
Agricultural soils covered by vegetation, crop residue or snow are partially protected and are less susceptible than bare soil to degradation processes such as wind and water erosion, organic matter depletion, structural degradation and loss of fertility. The amount of time soil is covered during a year depends on many factors such as the type of crop (perennial crops generally provide more cover than annual crops) and the amount of biomass it produces, the harvest practices used, the climate, and the type and timing of field operations, especially tillage practices. The Soil Cover Indicator summarizes the effective number of days in a year that agricultural soils are covered by vegetation, crop residue or snow. An increase in the number of soil cover days over time indicates an improvement in environmental sustainability since the soil is more protected from degradation and is less likely to contribute to water and air contamination.
The Soil Cover Indicator is estimated for each year since 1971, however this document will only discuss data correspondent to the census years between 1981 and 2016. Annual data results are available at Soil Cover Indicator. Over that 35-year period, average levels of soil cover in Canada have increased by 8.8%, from 261.7 to 284.7 days. This national scale improvement comes primarily as a result of widespread adoption of reduced (conservation) tillage and no-till, as well as decreases in the use of summer-fallow in Manitoba, Saskatchewan and Alberta. However, the improvement was offset to a considerable degree by shifts from relatively high-cover perennial crops to lower-cover annual crops nationally, especially crops with very low-cover such as potatoes, canola and soybeans.
The Issue and why it matters
Agricultural practices influence soil cover in many ways. The type of crop grown determines row spacing, growth rate, and the amount of biomass produced, and thus has a strong influence on the amount of soil cover produced in a given year. Perennial field crops such as hay offer good soil coverage year-round, while annual crops such as wheat or corn may leave soil exposed for a period after planting or after fall harvest and tillage. In addition, crops such as beans, peas, canola and potatoes tend to have a full canopy for shorter periods and leave lower residue levels after harvest. Residue management, including the method, timing and frequency of tillage, also has significant implications for soil cover. Intensive tillage (also referred to as conventional tillage) typically involves incorporating most of the crop residue into the soil to leave a clean surface for seeding, while reduced tillage and no-till leave more crop residue on the soil surface and thus provide greater cover. As well, tilling land in the fall leaves soil exposed for a greater length of time than tilling in the spring.
In agro-ecosystems, bare soil is more susceptible to degradation processes such as wind and water erosion, loss of organic matter, breakdown of soil structure and loss of fertility. The result of these degradation processes negatively impacts crop quality and yield. Degraded soil quality limits the type of crops that can be grown and requires more inputs, resulting in higher costs and reduced competitiveness to the producer. In extreme cases, soil degradation can result in the loss of agriculturally productive land. The issue of soil degradation is of concern not only from the perspective of soil quality, but also from a broader environmental perspective. Higher levels of erosion can increase the risk of contamination of ground and surface water by solids, nutrients and chemicals transported from agricultural fields. Bare soil also generally provides poor wildlife habitat and therefore can impact biodiversity.
Intensive tillage and removal of crop residue decrease soil cover directly. This leads to a reduction of carbon inputs to the soils, an increase of soil organic matter oxidation, hence a decrease of soil carbon sequestration and increase of CO2 emissions. Impact of tillage on CH4 and N2O fluxes is more complex and is dependent on soil properties. Compared to no-till, tilled soil potentially has a higher CH4 flux and a lower N2O flux (Mangalassery et al., 2014). Summer fallow has been a conventional dryland farming practice to restore soil moisture and fertility for cropping in the next year. However, this leaves soil susceptible to erosion. Replacing summer fallow with perennial crops or green manure crops such as lentils, and planting over-winter cover crops or intercropping, are now accepted beneficial practices to keep soil covered by plants on one hand, and to improve soil condition sequestration, suppress weeds growth, control pests, and enhance nutrients on the other hand.
The removal of crop residue (straw and stover) by burning or baling can have a negative effect on soil cover, but since burning is no longer a common practice in Canada and baling generally only occurs in areas where straw is plentiful, neither of these practices has a significant effect on national soil cover estimates. In addition, straw removed by baling is typically used as livestock bedding and much of this is eventually returned to the field with manure, thus limiting the overall and long-term effects of this management practice. Recent studies which involved modelling different residue harvest scenarios for bioenergy demonstrated that removing up to 40% of crop residue has little effect on soil cover, except in the Brown and Dark Brown soil zones in southern Saskatchewan and Alberta (Huffman et al., 2013, Liu et al., 2018). Currently, permanent removal of crop residue through baling or burning is generally restricted to flax fields in Manitoba, and the practice of burning straw has declined dramatically due to environmental concerns and improvements in the ability of field machinery to till and plant in heavy residue.
Exposed soils, particularly the Mollisol soils in the prairies, usually have a lower albedo than many crops and crop residues. Leaving these soils uncovered means less solar energy is reflected back to space, which modulates local energy balance, induces a warming effect and contributes to climate change (Liu et al., 2022).
The Indicator
The Soil Cover Indicator summarizes the effective number of days per year that agricultural soils are not exposed (Huffman et al., 2012, 2015). All land cover types under agricultural operations, which include land in crops, pasture land, and land used for other purposes in supporting of the farming operations, are considered in the indicator. The indicator considers soil cover provided by crop canopy, crop residues, and snow on the soil surface. One soil cover day (SCD) can be achieved with 100% cover for one day, 50% cover for two days, 10% cover for 10 days, and so on. Thus, the indicator reflects the annual effect of agricultural operations on soil cover. A perennial hay crop typically has more than 300 SCDs per year since there is very little soil exposed at any time. By contrast, a soybean crop in an area of low snowfall and with no winter cover crop may have less than 150 SCDs. The Soil Cover model evolves continuously over time as new data becomes available. For instance, Earth Observation (EO) products have been used to improve agricultural activity data previously available only through the agricultural census.
To estimate the number of SCDs, an annual calendar was developed that includes dates of typical field activities and soil cover amounts for each crop and tillage practices within each ecoregion. The Soil Cover Indicator takes into account the following variables:
- the days on which significant changes in soil cover occur (for example, planting, harvesting, tillage) and the percentage of soil cover upon completion of the operation,
- the duration (number of days) and percentage of soil cover between operations,
- canopy development and decline between planting and harvest,
- the decomposition of residue,
- the total number of days of snow cover greater than 2 cm,
- multiple cuts of hay and grazing on pasture.
A series of SCD calendars with appropriate crop growth and residue decomposition algorithms was developed for all crops and ecoregions in Canada using data from field studies (Wall et al., 2002), extension bulletins (Prairie Farm Rehabilitation Administration, 2003), published literature (for example, Steiner et al., 1999) and consultation with local agronomy experts. For crops with very limited extent, procedures were generated by extrapolating from known values for similar areas, crops and management practices. Thirty year climate normal data sets were used for daily weather for each ecoregion, rather than actual daily weather data, to keep weather variability from obscuring the impacts of practice change over time.
Crop areas and tillage distribution data at the Soil Landscape of Canada (SLC) polygon level were obtained from the EO-adjusted Census of Agriculture data for census years from 1981 through 2016. An area-weighted average SCD value was calculated for each SLC polygon, and aggregated to each province and for the whole country.
The indicator results are expressed in the mean annual number of SCDs at SLC level that can be aggregated to larger spatial scales, as well as the proportion of cropland, at the provincial level, falling into a distribution of five classes of soil cover days for each year evaluated. An increase in the number of soil cover days or in the proportion of land in the high cover classes over time suggests an improvement in sustainability and a declining likelihood that soils will become degraded or contribute to the degradation of the surrounding environment.
Limitations
A number of assumptions and limitations are inherent in the methodology. The use of 'typical' cropping practices and 30-year climate normals means that local (sub-ecoregion) variations in cropping practices, seeding and harvest dates and weather conditions are not accounted for. Also, since the Census of Agriculture is the only national source of tillage information and it does not distinguish differences in tillage practices amongst crops, the same distribution of intensive (conventional), reduced (conservation) and no-till is used for all crops within an SLC. Since conservation tillage and no-till have been used commonly only over the past 30 to 35 years and data on tillage practices has been collected in the census only since 1991, the conservation tillage and no-till rates are linearly interpolated (by region) back to zero, between 1991 and the year these practices were first adopted. These interpolated values are then used for 1981 and 1986.
Results and interpretation
Tillage practices, the frequency of perennial crop harvest, the use of summer-fallow, snow-cover and soil-climatic conditions vary across agricultural regions of Canada and these are reflected in the different average SCD values estimated for each region (Figure 1 and Table 1). The increased adoption of reduced tillage and no-till has had a positive influence on soil cover for all crops, in all regions of Canada and in all years under study (Figure 3). In the Prairie Provinces, adoption of reduced tillage and no-till and a decline in the frequency of summer-fallow contributed significantly to the increase in soil cover (Figure 2). Increases in the area of annual crops in eastern Canada (Figure 3), as well as national increases in low-cover annual crops such as canola, potatoes and soybeans, have negatively influenced soil cover over this period. For example, between 1981 and 2016, the percentage of agricultural land devoted to annual crops increased from 34.2% to 39.2% in Prince Edward Island, from 20.0% to 32.7% in Quebec, and from 40.1% to 51.6% in Ontario. During the same period, total area of canola increased by 6.9 MHa (from 1.4 to 8.3 MHa), and area of soybean increased by 2.0 MHa (from 0.3 to 2.3 MHa).
Table 1. Area-weighted mean annual soil cover days (SCD) by province and Canada, 1981-2016.
1981 | 1986 | 1991 | 1996 | 2001 | 2006 | 2011 | 2016 | |
---|---|---|---|---|---|---|---|---|
British Columbia | 286.3 | 296.0 | 297.7 | 300.4 | 301.7 | 304.4 | 302.2 | 301.4 |
Alberta | 267.0 | 270.5 | 272.3 | 276.8 | 283.2 | 289.0 | 290.7 | 289.1 |
Saskatchewan | 244.9 | 250.2 | 252.5 | 260.2 | 266.8 | 277.5 | 281.7 | 280.1 |
Manitoba | 263.3 | 268.6 | 270.7 | 273.6 | 276.6 | 282.3 | 284.3 | 278.7 |
Ontario | 268.9 | 272.9 | 271.8 | 276.4 | 276.3 | 277.8 | 276.4 | 273.5 |
Quebec | 309.5 | 311.9 | 309.9 | 310.1 | 306.7 | 307.9 | 309.9 | 308.9 |
New Brunswick | 325.2 | 327.7 | 326.0 | 327.3 | 324.9 | 325.9 | 329.4 | 329.3 |
Nova Scotia | 323.8 | 326.9 | 327.1 | 328.1 | 326.3 | 327.2 | 331.3 | 334.0 |
Prince Edward Island | 292.0 | 294.7 | 293.3 | 290.2 | 289.0 | 289.5 | 288.9 | 289.7 |
Newfoundland and Labrador | 298.0 | 329.9 | 327.4 | 334.9 | 332.3 | 319.8 | 324.3 | 326.0 |
Canada | 261.7 | 266.0 | 267.2 | 272.6 | 277.3 | 284.3 | 286.8 | 284.7 |
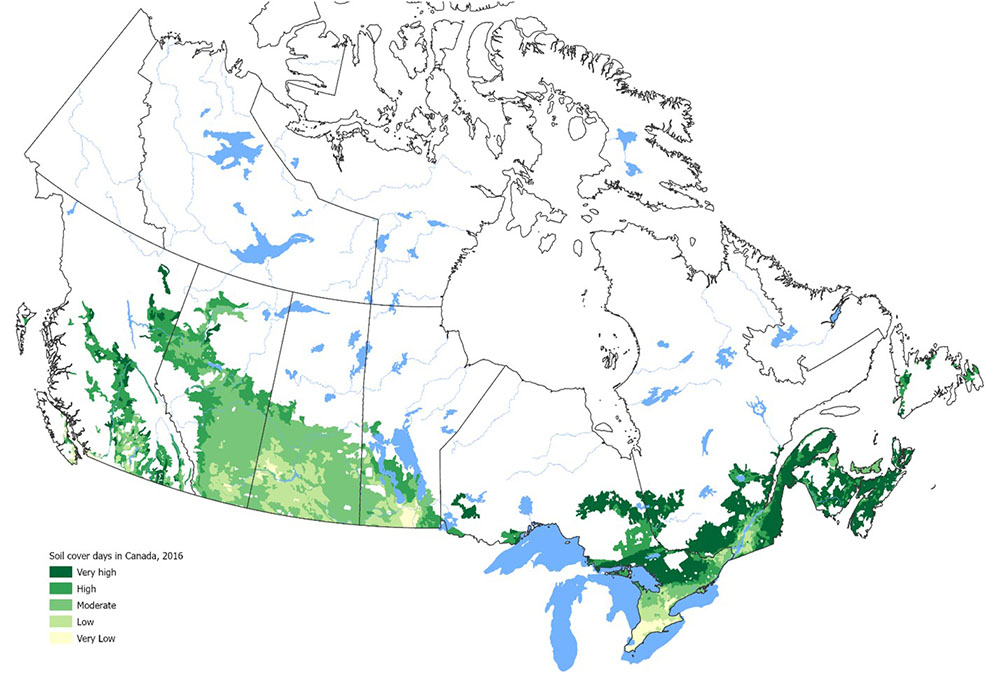
Figure 1. Soil cover days in Canada, 2016, by soil landscape unit, Canada
Description of Figure 1
Figure 1 illustrates the soil cover days in Canada for 2016, color coded based on the number of soil cover days.
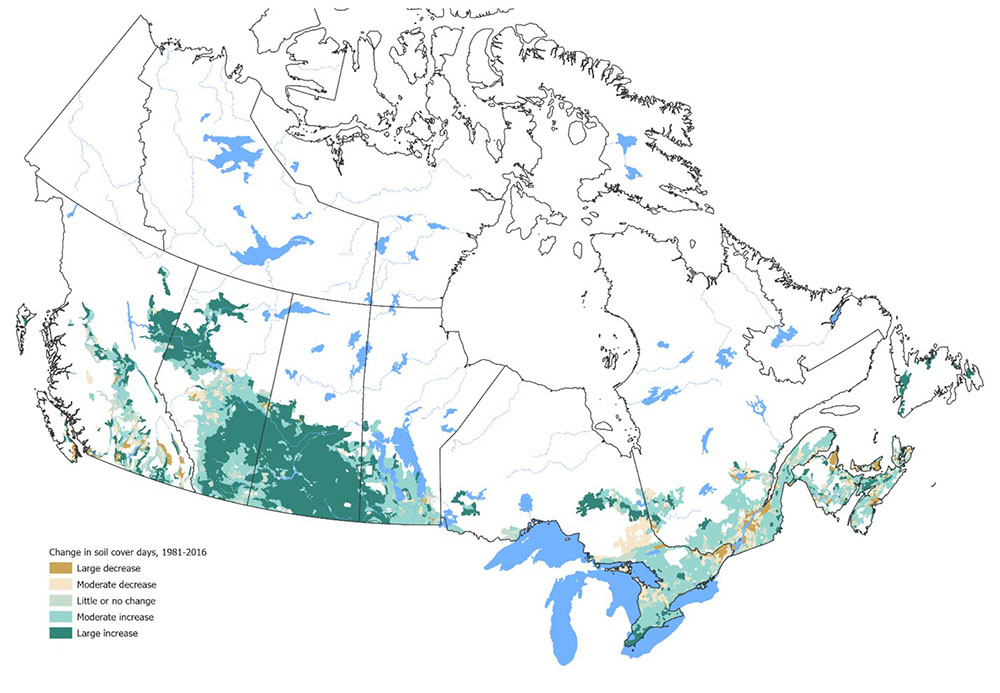
Figure 2. Change in soil cover days, 1981-2016, by soil landscape unit, Canada
Description of Figure 2
Figure 2 illustrates the change soil cover days in Canada between 1981 and 2016, color coded based on whether the number of soil cover days decreased or increased or did not change.
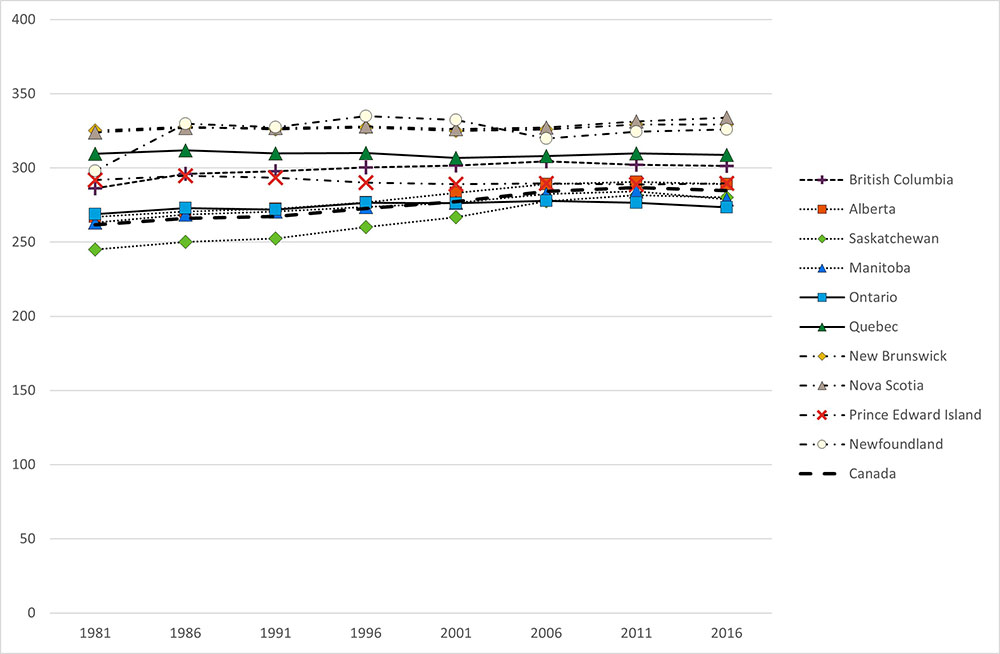
Figure 3. Area-weighted annual mean soil cover days (SCD) by province and Canada, 1981-2016_EN_r_2024-05-13
Description of Figure 3
Area-weighted mean annual soil cover days (SCD), 1981-2016
1981 | 1986 | 1991 | 1996 | 2001 | 2006 | 2011 | 2016 | |
---|---|---|---|---|---|---|---|---|
British Columbia | 286.3 | 296.0 | 297.7 | 300.4 | 301.7 | 304.4 | 302.2 | 301.4 |
Alberta | 267.0 | 270.5 | 272.3 | 276.8 | 283.2 | 289.0 | 290.7 | 289.1 |
Saskatchewan | 244.9 | 250.2 | 252.5 | 260.2 | 266.8 | 277.5 | 281.7 | 280.1 |
Manitoba | 263.3 | 268.6 | 270.7 | 273.6 | 276.6 | 282.3 | 284.3 | 278.7 |
Ontario | 268.9 | 272.9 | 271.8 | 276.4 | 276.3 | 277.8 | 276.4 | 273.5 |
Quebec | 309.5 | 311.9 | 309.9 | 310.1 | 306.7 | 307.9 | 309.9 | 308.9 |
New Brunswick | 325.2 | 327.7 | 326.0 | 327.3 | 324.9 | 325.9 | 329.4 | 329.3 |
Nova Scotia | 323.8 | 326.9 | 327.1 | 328.1 | 326.3 | 327.2 | 331.3 | 334.0 |
Prince Edward Island | 292.0 | 294.7 | 293.3 | 290.2 | 289.0 | 289.5 | 288.9 | 289.7 |
Newfoundland | 298.0 | 329.9 | 327.4 | 334.9 | 332.3 | 319.8 | 324.3 | 326.0 |
Canada | 261.7 | 266.0 | 267.2 | 272.6 | 277.3 | 284.3 | 286.8 | 284.7 |
Provincial level SCD is given in Table 1. It is mainly determined by typical regional farming practices. The prairie provinces generally have a larger proportion of farmland under annual crops than the Atlantic provinces (NL, NS and NB) hence a relatively lower SCD. Provincial average SCD values over the period from 1981 to 2016 ranged from a low of 245 in Saskatchewan in 1981 to a high of 334.0 in Nova Scotia in 2016 (Table ). The national average increased over the 35-year period from 261.7 to 284.7 (Table 1), with the greatest increases occurring in Saskatchewan (14.4%), Newfoundland and Labrador (9.4%), Alberta (8.3%), Manitoba (5.8%) and British Columbia (5.3%). Smaller increases occurred in Nova Scotia (3.2%), Ontario (1.7%) and New Brunswick (1.2%), while average SCD decreased by about 0.8% in Prince Edward Island and 0.2% in Quebec. The national increase in SCD over this period was driven primarily by the prairie provinces by virtue of their large agricultural area and relatively large increases in soil cover as a result of reductions in tillage and summer-fallow area.
In order to demonstrate the spatial variability in soil cover across the country, we defined 'very high' soil cover as being greater than 325 days per year, 'high' as 300-324 days, 'moderate' as 275-299 days, 'low' as 250-274 days and 'very low' as fewer than 250 days. The proportion of farmland in each of these classes by province and by census year is presented in Table 2, and the spatial distribution of the indicator, calculated on the basis of Soil Landscapes of Canada polygons, is shown in Figure 1. About 3.0% of Canadian farmland was in the very high soil cover class in 1981, and increased to 3.3% by 2016, while the proportion of land in the high soil cover class rose from 6.2% in 1981 to 11.1% in 2016 and the proportion in the moderate class rose from 18.8% in 1981 to 62.4% in 2016. These increases were the result of a decrease in the proportion of land in both the low (from 41.8% to 20.8%) and very low classes (from 30.2% to 2.4%). Provinces with the highest percentage in the very high class include Newfoundland and Labrador (61% in 2016), Quebec (37%), New Brunswick (59%) and Nova Scotia (79%). Ontario had a higher proportion in the very low soil cover class all the years, with 25% of agricultural land in this category in 2016, while Saskatchewan showed the most change, improving steadily from 52% of lands in the very low soil cover class in 1981 to 0% in 2016.
Table 2. Percentage of farmland by soil cover classes, 1981-2016.
Census year | B.C. | Alta. | Sask. | Man. | Ont. | Que. | N.B. | N.S. | P.E.I. | N.L. | Can. | |
---|---|---|---|---|---|---|---|---|---|---|---|---|
Very high (>=325 days) | 1981 | 0 | 0 | 0 | 0 | 2 | 34 | 57 | 68 | 0 | 0 | 3 |
1986 | 2 | 0 | 0 | 0 | 4 | 39 | 67 | 75 | 0 | 64 | 4 | |
1991 | 2 | 0 | 0 | 0 | 4 | 36 | 65 | 73 | 0 | 27 | 3 | |
1996 | 3 | 0 | 0 | 0 | 6 | 38 | 69 | 73 | 0 | 82 | 4 | |
2001 | 4 | 0 | 0 | 0 | 6 | 37 | 62 | 67 | 0 | 84 | 3 | |
2006 | 7 | 0 | 0 | 0 | 4 | 31 | 72 | 78 | 0 | 20 | 3 | |
2011 | 5 | 0 | 0 | 0 | 5 | 39 | 62 | 74 | 0 | 40 | 3 | |
2016 | 8 | 0 | 0 | 0 | 4 | 37 | 57 | 79 | 0 | 54 | 3 | |
High (300~325 days) | 1981 | 35 | 3 | 0 | 3 | 14 | 38 | 39 | 22 | 23 | 45 | 6 |
1986 | 50 | 6 | 0 | 5 | 15 | 33 | 27 | 17 | 29 | 36 | 7 | |
1991 | 54 | 6 | 0 | 4 | 17 | 37 | 29 | 18 | 25 | 70 | 8 | |
1996 | 62 | 9 | 0 | 8 | 19 | 33 | 26 | 22 | 14 | 18 | 9 | |
2001 | 63 | 13 | 0 | 7 | 15 | 27 | 26 | 22 | 0 | 16 | 9 | |
2006 | 63 | 17 | 2 | 8 | 21 | 36 | 21 | 20 | 11 | 78 | 12 | |
2011 | 64 | 14 | 2 | 8 | 12 | 28 | 31 | 21 | 1 | 56 | 10 | |
2016 | 57 | 12 | 1 | 5 | 11 | 28 | 37 | 17 | 1 | 45 | 8 | |
Moderate (275~300 days) | 1981 | 40 | 30 | 7 | 11 | 33 | 17 | 4 | 8 | 63 | 55 | 18 |
1986 | 29 | 33 | 9 | 16 | 31 | 16 | 5 | 6 | 56 | 0 | 20 | |
1991 | 33 | 38 | 11 | 19 | 30 | 16 | 6 | 10 | 57 | 3 | 23 | |
1996 | 24 | 43 | 18 | 24 | 27 | 16 | 6 | 3 | 68 | 0 | 27 | |
2001 | 20 | 64 | 31 | 37 | 31 | 18 | 12 | 9 | 85 | 0 | 41 | |
2006 | 20 | 69 | 56 | 56 | 28 | 21 | 7 | 1 | 85 | 2 | 54 | |
2011 | 16 | 76 | 67 | 56 | 35 | 19 | 7 | 2 | 97 | 4 | 62 | |
2016 | 19 | 77 | 67 | 46 | 32 | 20 | 6 | 3 | 99 | 1 | 61 | |
Low (250~275 days) | 1981 | 17 | 51 | 43 | 62 | 20 | 12 | 0 | 2 | 14 | 0 | 42 |
1986 | 14 | 47 | 51 | 61 | 20 | 11 | 0 | 2 | 14 | 0 | 44 | |
1991 | 5 | 43 | 51 | 59 | 19 | 11 | 0 | 0 | 18 | 0 | 42 | |
1996 | 5 | 39 | 56 | 50 | 23 | 13 | 0 | 1 | 18 | 0 | 43 | |
2001 | 6 | 19 | 57 | 39 | 23 | 19 | 0 | 2 | 15 | 0 | 36 | |
2006 | 5 | 13 | 41 | 20 | 29 | 13 | 0 | 1 | 3 | 0 | 26 | |
2011 | 9 | 10 | 31 | 20 | 27 | 15 | 0 | 3 | 2 | 0 | 21 | |
2016 | 9 | 11 | 31 | 34 | 29 | 15 | 0 | 0 | 0 | 0 | 23 | |
Very low (>=250 days) | 1981 | 8 | 16 | 50 | 24 | 31 | 0 | 0 | 0 | 0 | 0 | 30 |
1986 | 5 | 14 | 40 | 18 | 30 | 0 | 0 | 0 | 0 | 0 | 25 | |
1991 | 6 | 13 | 39 | 17 | 31 | 0 | 0 | 0 | 0 | 0 | 24 | |
1996 | 6 | 10 | 26 | 18 | 25 | 0 | 0 | 0 | 0 | 0 | 18 | |
2001 | 6 | 4 | 11 | 17 | 25 | 0 | 0 | 0 | 0 | 0 | 10 | |
2006 | 4 | 2 | 1 | 15 | 18 | 0 | 0 | 0 | 0 | 0 | 4 | |
2011 | 7 | 0 | 0 | 15 | 21 | 0 | 0 | 0 | 0 | 0 | 4 | |
2016 | 7 | 0 | 1 | 15 | 24 | 0 | 0 | 0 | 0 | 0 | 5 |
The national spatial distribution of changes in soil cover between 1981 and 2016 is shown in Figure 2. Most of the central prairie region, where reductions in tillage and summer-fallow area have been significant, has seen increases of more than 10 days, while moderate increases were seen in the agricultural fringe areas with more perennial crops, where SCDs were already higher and there was less opportunity for reducing tillage and decreasing summer-fallow levels. The majority of the remaining agricultural regions of the country have undergone moderate to slight changes, either decreases or increases. In contrast, several areas in southern British Columbia and the St Lawrence Lowlands in eastern Ontario and western Quebec showed decreases of greater than 10 days. These areas underwent significant changes in crop distribution, with relatively large shifts from perennial to annual crops during the period under study.
Response options
Changes in average soil cover within a region are influenced by changes in both tillage practices (intensive vs. reduced vs. no-till) and crop distribution (high vs. low residue, annual vs. perennial). Thus, although the adoption of reduced tillage may increase soil cover for a specific crop, a shift from intensive tillage on a high-residue crop such as cereal grain to reduced tillage on a lower-residue crop such as canola could result in a decrease in SCDs.
Of perhaps even greater importance than the adoption of reduced tillage in improving soil cover is the application of practices to enhance soil cover during the production of annual and inherently low-residue crops such as potatoes, canola, soybeans, vegetables and nursery crops. These crops show an increase in area since 1981 and can be expected to continue to expand (Statistics Canada, 2016). Planting a green manure or winter cover crop where feasible as soon as possible after harvesting these crops would provide a greater degree of soil cover during the long period between harvesting in the fall and planting in the spring. This may become especially important if climatic changes reduce the number of days of soil protection afforded by snow or if extreme weather events become more common in the spring before planting, when the soil is particularly vulnerable to degradation through erosion. The desire for greater soil cover also has some research implications in the development of intercropping systems, and the development of cold-germination varieties of crops for use under no-till, in the development of equipment to better maintain surface residue while performing production operations satisfactorily and perhaps even in the development of crops with a greater mass of more durable foliage.
Since the early 1980s, the trend in the level of soil cover days has been generally positive. However, the increase in soil cover has slowed almost nationally from a high rate of change in the early to mid-1990s to much more modest increases since then. This is indicative of the increasing technical challenges as the rate of adoption of reduced tillage practices is reaching a plateau and further expansion may not keep up with the negative influence of cropping system changes, such as increased use of crops with low residue production, in particular canola and soybeans. Anecdotal evidence suggests that the high amount of stover associated with high-yielding corn, and the resistance to degradation of corn stover may be increasing the need for more intensive tillage practices. It is also noted that harvesting of more than 40% of crop residue, which could become a reality under a strong bio-economy, can have a deleterious effect on soil cover (Liu et al., 2018). Thus, the adoption of practices to mitigate losses of soil cover, such as winter cover crops, intercropping, and residue management, are essential for offsetting these cropping system changes and maintaining increases in soil cover.
References
- Huffman, T., Liu, J., Green, M., Coote, D., Li, Z., Liu, H., Liu, T., Zhang, X., Du, Y. 2015. Improving and evaluating the soil cover indicator for agricultural land in Canada. Ecological Indicators, 48:272-281.
- Huffman, T., Liu, J., Coote, D and Green, M. 2013. The effect on soil cover of integrating perennial crops into cropping systems in response to demand for bioenergy feedstock. ASA/CSSA/SSSA International Annual Meetings, Tampa, FL, Nov 3-6, 2013. Invited oral presentation.
- Huffman, T., Coote, D.R. and Green, M. 2012. Twenty-five years of changes in soil cover on Canadian Chernozemic (Mollisol) soils, and the impact on the risk of soil degradation. Canadian Journal of Soil Science. 92:471-479.
- Liu, J., Huffman, T., Green, M. 2018. Potential impacts of agricultural land use on soil cover in response to bioenergy production in Canada. Land Use Policy, 75:33-42.
- Liu, J., Desjardins, R.L., Wang, S., Worth, D.E., Qian, B., Shang, J. 2022. Climate impact from agricultural management practices in the Canadian Prairies: Carbon equivalence due to albedo change. Journal of Environmental Management, 302, art.# 113938.
- Mangalassery, S., Sjögersten, S., Sparkes, D.L., Sturrock, C.J., Craigon, J., Mooney, S.J., 2014. To what extent can zero tillage lead to a reduction in greenhouse gas emissions from temperate soils? Scientific Reports 4: 4586.
- National Land and Water Information Service. 2008. Interpolated census of agriculture to soil landscapes, ecological frameworks and drainage areas of Canada. Retrieved April 27, 2021 from: Interpolated Census of Agriculture by Soil Landscapes of Canada.
- Prairie Farm Rehabilitation Administration. 2003. Managing crop residues on the prairies. Internal archives. About the census of agriculture. Retrieved June 25, 2008 from: Census of Agriculture
- Statistics Canada, 2016. 2016 Census of Agriculture. Retrieved February 24, 2022 at: 2016 Census of Agriculture.
- Statistics Canada, 2016. 2016 Census of Agriculture.
- Steiner, J.L., Schomberg, H.H., Unger, P.W., and Cresap, J., 1999. Crop residue decomposition in no-tillage small-grain fields. Soil Science Society of America Journal. 63:1817-1824.
- Wall, G.J., Coote, D.R., Pringle, E.A. & Shelton, I.J. Eds. 2002. RUSLEFAC - Revised universal soil loss equation for application in Canada: A handbook for estimating soil loss from water erosion in Canada. Ottawa, ON, Canada: Agriculture and Agri-Food Canada.
- Wischmeier, W.H. & Smith, D.D. 1965. Predicting rainfall erosion loss from cropland east of the Rocky Mountains in Agriculture Handbook No. 282. Washington, DC: USDA.